|
|
Genetic Analysis of Variation in Neuron Number Richelle Cutler
Strom
Chapter 5: Mapping Genes
Controlling Variation in Retinal Ganglion Cell Number Using Recombinant
Inbred Strains and F2 Intercross Progeny
Introduction
The high heritability of retinal ganglion cell number among mouse strains
indicates that the variation in ganglion cell number results primarily from
genetic differences. Furthermore, the prominent bimodality in ganglion cell
number among 57 inbred strain averages demonstrates that only a few genes
with major effects are responsible for variation in ganglion cell number.
Together, these findings suggest that it should be possible to map QTLs that
are responsible for variation in ganglion cell number between strains. Only
one other study has mapped QTLs controlling neuron number in mice. This
study, by Dains et al. (1994), examined variation in the number of striatal
cholinergic neurons among inbred mouse strains. A greater than 50% variation
was found between BXD strains. The narrow-sense heritability in the BXD
strains was found to be around 0.30 and the number of effective factors was
from 3 to 4. Using BXD strains, QTLs associated with variation in
cholinergic neuron number were mapped to chromosomes 1, 6, 9, 11 and 12.
In this study, I use BXD recombinant inbred strains and two F2
intercrosses in a linkage analysis to map QTLs that produce variation in
retinal ganglion cell number. In order to map genes that modulate ganglion
cell number directly, rather than genes that modulate global neuron number,
I have mapped using ganglion cell number residuals regressed with respect to
brain weight. Thus, the QTLs that are detected are expected to have specific
effects on the ganglion cell population.
Variation in neuron number
After detecting significant linkage between a QTL interval and variation
in ganglion cell number, the next step is to look for candidate genes within
the QTL interval. There are two criteria for selecting candidate genes that
map within the QTL interval. The first is that it is expressed during
prenatal or early postnatal eye development, and the second is that it has
an assigned function consistent with a role in cell production or cell
death. In this study, I examine two candidate genes that meet these
criteria. The candidate genes were examined by comparing ganglion cell
numbers in wild type mice to those in mice carrying a nonfunctional
transgene at the candidate locus.
Materials and methods
The BXD recombinant inbred strains were used to
map genes modulating ganglion cell number. Two F2
intercrosses were then used to confirm QTLs mapped in the BXD RI strains and
to map additional QTLs. I generated two groups of intercross progeny from
matings between inbred strains BALB/cJ and CAST/Ei (CCASF2,
n =112), and BXD32 and CAST/Ei (32CASF2,
n =140). The parental strains BALB/cJ, BXD32 and CAST/Ei have ganglion
cell numbers of 63,400, 75,800, and 45,000, respectively. To perform a
genome-wide linkage analysis DNA was genotyped from CCASF2
and 32CASF2 individuals at 110 microsatellites.
Candidate genes were tested by comparing ganglion cell counts from mice
containing a nonfunctional transgene at the candidate locus to ganglion cell
counts from mice carrying a normal functional candidate. Two candidate genes
were tested, retinoic acid receptor alpha 1 (Rara1) and
thyroid receptor alpha (Thra). The Rara1 null mice were
obtained from Vincent Giguere at Royal Victoria Hospital in Montreal,
Canada, while the Thra null mice were obtained from Douglas Forrest
at Mount Sinai in New York. The homozygous Rara1 null mice appeared
completely normal, whereas the Rara null mice had high postnatal
lethality and testis degeneration (Lufkin et al., 1993). The homozygous
Thra null mice have a 20% lower heart rate and a 0.5 °C lower body
temperature, but otherwise are healthy and fertile (Wikstrom et al., 1998).
The examination of the Rara1 and Thra knock-out mice
described here is preliminary work carried out as part of a collaboration
with Drs. Vincent Giguere and Douglas Forrest. The preliminary work on
Rara1 has been published (Zhou et al. 1998). Final publications are in
progress (Zhou G, Strom R, Giguere V, Williams RW).
Results
The average ganglion cell number in the BXD strains ranges from 52,500 in
BXD27 to 76,200 in BXD32. There are 8 strains with
low ganglion cell means and 14 strains with high ganglion cell means. In
Table 5.1, the low strains are assigned L and the high strains are assigned
H. There are five strains with intermediate ganglion cell numbers that fall
within ± 2,000 of the mid-parental value of 59,000 and these strains are
assigned an I in Table 5.1.
Table 5.1 Corrected retinal ganglion cell
number for BXD strains and parents, C57BL/6J and DBA/2J.
Strain |
RGC |
± SE |
Type |
n |
Brain weight |
C57BL/6J |
55,413 |
± 800 |
L |
26 |
475 |
DBA/2J |
63,351 |
± 1,200 |
H |
13 |
412 |
BXD1 |
58,714 |
± 1,058 |
I |
11 |
465 |
BXD2 |
65,790 |
± 1,562 |
H |
6 |
432 |
BXD5 |
71,228 |
± 1,162 |
H |
6 |
526 |
BXD6 |
64,275 |
± 805 |
H |
6 |
388 |
BXD8 |
60,913 |
± 2,092 |
I |
8 |
412 |
BXD9 |
65,449 |
± 1,883 |
H |
3 |
422 |
BXD11 |
60,734 |
± 1,013 |
I |
9 |
437 |
BXD12 |
56,256 |
± 1,421 |
L |
2 |
434 |
BXD13 |
54,869 |
± 1,491 |
L |
7 |
427 |
BXD14 |
63,507 |
± 1,435 |
H |
7 |
442 |
BXD15 |
63,246 |
± 973 |
H |
6 |
443 |
BXD16 |
62,294 |
± 1,124 |
I |
6 |
469 |
BXD18 |
55,151 |
± 1,192 |
L |
4 |
427 |
BXD19 |
67,009 |
± 1,200 |
H |
9 |
431 |
BXD20 |
61,312 |
± 1,728 |
I |
10 |
398 |
BXD21 |
59,383 |
± 1,330 |
I |
8 |
443 |
BXD22 |
63,244 |
± 944 |
H |
5 |
457 |
BXD23 |
52,797 |
± 911 |
L |
6 |
434 |
BXD24 |
64,150 |
± 918 |
H |
7 |
391 |
BXD25 |
53,778 |
± 1,314 |
L |
7 |
431 |
BXD27 |
52,483 |
± 973 |
L |
6 |
393 |
BXD28 |
53,430 |
± 1,644 |
L |
7 |
407 |
BXD29 |
64,350 |
± 1,182 |
H |
6 |
413 |
BXD30 |
67,429 |
± 1,137 |
H |
6 |
399 |
BXD31 |
66,733 |
± 1,053 |
H |
6 |
426 |
BXD32 |
76,219 |
± 1,914 |
H |
10 |
434 |
A comparison of ganglion cell number in the 26 BXD strains and the
genotypic strain distribution at 529 loci resulted in the best match at
Tstap91A located on Chr 11 at 75 cM. A correlation between
alleles at Tstap91A and ganglion cell number resulted in a
coefficient of +0.72. The LOD score for the linkage between ganglion cell
number and Tstap91A is 4.5. Table 5.2 shows the concordance between
the genotypic strain distribution of loci on Chr 11 and the putative
genotype at the RGC locus based on its parental resemblance. There are 20
strains with RGC phenotypes concordant with alleles at Tstap91A,
while only 1 strain is discordant and 5 strains are intermediate.
Table 5.2. BXD strain distribution pattern for
retinal ganglion cell number and genotypes at five loci on Chr 11.
Locus |
cM |
BXD strains |
|
|
1 |
2 |
5 |
6 |
8 |
9 |
11 |
12 |
13 |
14 |
15 |
16 |
18 |
19 |
20 |
21 |
22 |
23 |
24 |
25 |
27 |
28 |
29 |
30 |
31 |
32 |
D11Ncvs58 |
56 |
B |
D |
D |
B |
D |
D |
B |
D |
B |
D |
D |
D |
B |
D |
D |
D |
D |
B |
B |
B |
D |
B |
D |
B |
B |
D |
Hoxb |
56 |
B |
D |
D |
D |
D |
D |
B |
B |
B |
D |
D |
D |
B |
D |
D |
D |
D |
B |
D |
B |
D |
B |
D |
D |
B |
D |
RGC number* |
|
I |
D |
D |
D |
I |
D |
I |
B |
B |
D |
D |
I |
B |
D |
I |
I |
D |
B |
D |
B |
B |
B |
D |
D |
D |
D |
Tstap91A |
57 |
B |
D |
D |
D |
D |
D |
B |
B |
B |
D |
D |
D |
B |
D |
D |
D |
D |
B |
D |
B |
B |
B |
D |
D |
B |
D |
Krt1 |
58 |
B |
D |
D |
D |
D |
D |
B |
B |
B |
D |
D |
D |
B |
D |
D |
D |
D |
B |
D |
B |
B |
B |
B |
D |
B |
D |
Mpmv8 |
62 |
B |
D |
D |
D |
D |
D |
B |
B |
B |
D |
D |
B |
D |
D |
D |
D |
D |
B |
D |
B |
B |
B |
B |
D |
B |
D |
*The alleles for RGC number are
based on the strain’s resemblance to the C57Bl/6J (B) or DBA/2J (D)
phenotype. The B allele was assigned if ganglion cell number was <57,000 and
the D allele was assigned if the ganglion cell number was >61,000. Strains
with ganglion cell numbers >57,000 but <61,000 were assigned an intermediate
(I) allele type.
Controlling for the Tstap91A indicated secondary loci on distal
Chr 1 at D1Ncvs60 and on proximal Chr 7 at Iapls3-4;1-11.
Controlling for these two secondary loci increased the LOD of Tstap91A
to 6.7 at 57 cM, with a 2 LOD CI between Hoxb and Krt1, at 56
and 58 cM, respectively (Fig 5.1). The second close match with ganglion cell
number was found at D14Pas1 on Chr 14 at 12 cM, with a correlation
coefficient of +0.60 and an LOD score of 3.5. A linkage test while
controlling for the variance at D14Pas1 detected secondary loci on
Chr 2 at D2Byu2 and on Chr 6 at D6Mit86. Interval mapping
while controlling for these two secondary loci increased the LOD at
D14Pas1 to 5.9 at 15 cM, with a 2 LOD confidence interval from 11 to 17
cM (Fig 5.2).
A permutation test in the BXD data set while controlling for secondary
loci defined the appropriate genome-wide conditional significance
thresholds. The LOD scores required for a suggestive, significant and highly
significant linkage are 3.0, 4.7, and 6.0, respectively. The QTLs detected
on Chr 11 with peak LOD score of 6.7 exceeds the highly significant
threshold. The locus on proximal Chr 14 with a peak LOD score of 5.9, almost
reaches the highly significant threshold. The QTL mapped to Chr 11 has been
named Neuron number control 1 (Nnc1) (Williams et al., 1998),
and the QTL mapped to Chr 14 has been named Neuron number control 2 (Nnc2).
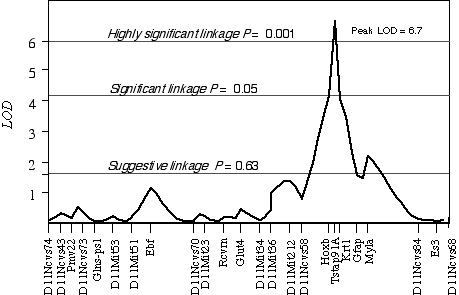
Figure 5.1. Linkage map demonstrates the QTL Nnc1 on Chr 11
in the BXD data set. LOD scores were computed at 1 cM intervals while
controlling for Iapls3-4; 1-11 on Chr 7 and D1Ncvs60 on Chr 1.
My analysis detected a significant linkage on Chr 14 that was not
reported in Williams et al., (1998). A possible explanation for the
additional linkage is a difference in the regression procedure. In this
proceeding, I truncated the ganglion cell numbers in the BXD5 and BXD32
strains before the regression of ganglion cell number and brain weight. I
did this because the ganglion cell numbers for BXD5 and BXD32 exhibited
overdominance and in the case of BXD5 also an overdominance in brain weight.
In a regression analysis, a large deviation in just a few data points can
distort the true association. The overdominance in these strains may result
from unique non-additive epistatic interactions or a combination of genes
with increaser alleles, which could produce a global increase in cell
number, and may interfere with linkage associations.
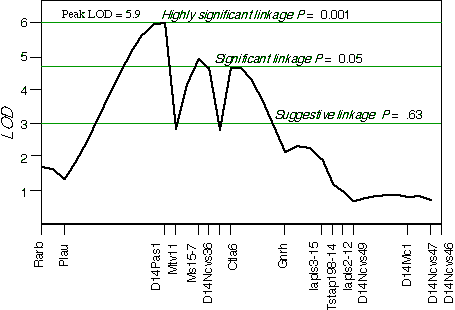
Figure 5.2.Linkage map demonstrates the QTL Nnc2 on Chr 14 in
the BXD data set. LOD scores were computed at 1 cM intervals while
controlling for D2Byu2 on Chr 2 and D6Mit86 on Chr 6.
Testing Nnc1 candidate genes by examining knock out mice
Three excellent candidates for Nnc1 that map within the Hoxb—Krt1
interval, retinoic acid receptor alpha 1 (Rara1), thyroid
receptor alpha (Thra), and the neuregulin receptor Erbb2.
All three of these receptors are expressed in the developing retina and the
ligands for these receptors are known to influence retinal cell number (Bermingham-McDonogh
et al., 1996; Ruberte et al., 1993; Sjoberg et al., 1992). The addition of
neuregulin, the ligand that activates the receptor Erbb2, promotes
ganglion cell survival in culture (Bermingham-McDonogh et al., 1996). The
addition of retinoic acid and thyroid hormone to retinal cultures increases
the differentiation of photoreceptors (Kelley et al., 1995). A reciprocal
relationship in differentiation potential is thought to occur between the
early- and late-generated retinal cell types. Thus, an increased bias for
the generation of ganglion cells could have repercussions on the number
later-generated photoreceptors.
The average ganglion cell number in the transgenic mice carrying a null
Rara1 is 62,494 ± 1160 (n = 10) compared to the average for
normal Rara1 mice of 62,998 ± 1930 (n = 9) (Fig. 5.3A). There
are no significant differences in ganglion cell number between the
homozygous mutant Rara1 and the normal Rara1 mouse, p =
0.82. In contrast, the average number of ganglion cells in the transgenic
mice carrying a null Thra1 is 63,645 ± 1884 (n = 9), compared
to an average of 74,030 ± 1137 (n = 8) in mice carrying a normal
Thra1 (Fig. 5.3B). The difference in ganglion cell number between the
homozygous null and normal Thra mice is highly significant at p
= 0.0005.
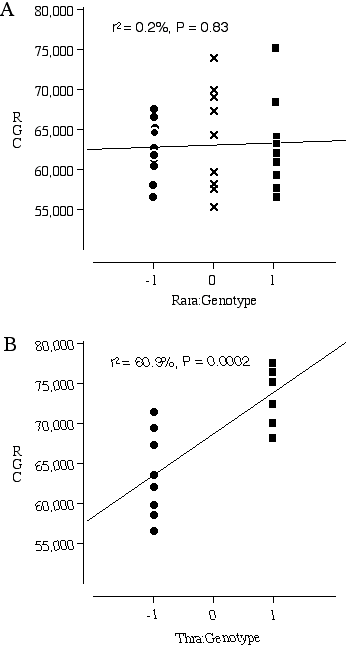
Figure 5.3. Ganglion cell numbers for transgenic mice carrying
homozygous null Nnc1 candidate genes (–1), heterozygous null (0) and
wildtype genes (1). (A) Rara1
(–/–)(–/+)(+/+) comparison; (B) Thra (–/–)(+/+) comparison.
In CCASF2 mice, ganglion cell number corrected
with respect to brain weight ranged from 41,000 to 70,000. I performed a
linkage analysis between ganglion cell number and the genotypes of 30
extreme CCASF2 progeny. The best association with
ganglion cell number was on distal Chr 1 at D1Mit105 with a LOD score
of 3.5. The second best linkages were on proximal Chr 15 at D15Mit12
with a LOD score of 3.2 and on mid-distal Chr 7 at D7Mit238 with a
LOD score of 2.3. Linkage testing while controlling for the Chr 1 locus
detected a linkage on Chr 16 near D16Mit29. Composite interval
mapping on Chr 1 while controlling for the QTL intervals on Chrs 7, 15, and
16 resulted in an LOD score of 9.3 between D1Mit502 and D1Mit105.
Linkage testing while controlling for D15Mit12 detected a linkage on
Chr 10 near D10Mit282 with a LOD score of 2.7. Interval mapping while
controlling for the QTLs on Chrs 1, 7, and 10 increased the LOD at
D15Mit12 to 4.0.
Increasing the number of progeny in a linkage analysis can improve the
estimate of the QTL location. Interval mapping on Chr 1 with 100 CCASF2
while controlling for D15Mit12 and D13Mit18 resulted in an LOD
of 6.0 between D1Mit105 and D1Mit57, with the peak score at 82
cM, 2 cM distal of D1Mit105 (Fig. 5.4). The 2 LOD CI is from 77 cM to
88 cM. Variation at D1Mit105 is estimated to explain 15% of
variance in cell number, however, estimates in small sample sizes such as
this one, tend to overestimate the effect size. Interval mapping on Chr 15
with 100 CCASF2 and controlling for D1Mit105
resulted in an LOD of 2.0 at D15Mit12, the most proximal marker.
A permutation test with the complete data set while controlling for
secondary loci defined the genome-wide significance thresholds. The LOD
scores required for a suggestive, significant and highly significant linkage
are 2.0, 3.5, and 5.4, respectively. The LOD score reached 2.0 at
D15Mit12 and is therefore only a suggestive linkage. The LOD score in
the interval between D1Mit105 and D1Mit57 reaches 6.0, and is
a highly significant linkage. I have named the QTL on distal Chr 1 neuron
number control 3 (Nnc3).
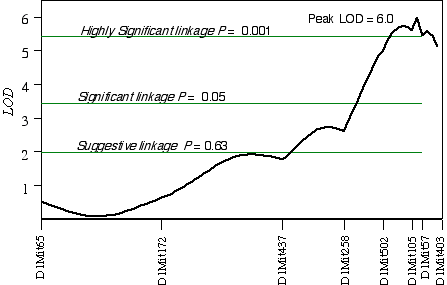
Figure 5.4.
Linkage map demonstrates the QTL Nnc3 on distal Chr 1 in the CCASF2
data set. LOD scores were computed at 1 cM intervals while controlling
for D15Mit12 and D13Mit18.
In 32CASF2 mice, ganglion cell number corrected
with respect to brain weight ranged from 41,000 to 70,000. A comparison
between ganglion cell number and genotypes in the 45 extreme 32CASF2
progeny detected a linkage on mid-Chr 16 at D16Mit190 with a LOD
score of 2.6. A linkage test while controlling for the D16Mit190
resulted in detecting secondary loci D13Mit203 and D17Mit10.
Composite interval mapping on Chr 16 while controlling for D13Mit203
and D17Mit10 increased the LOD score to 6.0 at D16Mit190,
which maps at 41.5 cM (Fig. 5.5). The 2 LOD CI is from 22 cM to 50 cM. A
permutation test defined the appropriate genome-wide significance
thresholds. The LOD scores required for a suggestive, significant and highly
significant linkage are 2.0, 3.6, and 5.9, respectively. The linkage at
D16Mit190 with a LOD score of 6.0 is therefore a highly significant
linkage. I have named the QTL on Chr 16 neuron number control 4 (Nnc4).
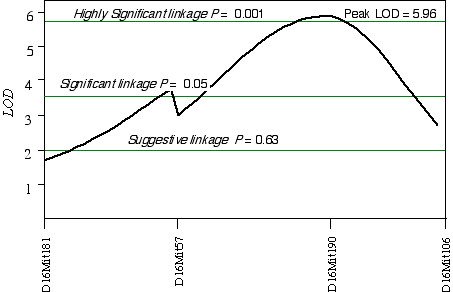
Figure 5.5. Linkage map demonstrates Nnc4 on Chr 16 in the
32CASTF2 data set.
LOD scores were computed at 1 cM intervals while controlling for
D13Mit203 and D17Mit10.
Discussion
Synopsis
I have mapped four significant QTLs that modulate ganglion cell number in
mice to Chr 1, 11, 14, and 16. These QTLs have been named Nnc1, 2, 3,
and 4. Nnc1 maps to 57 cM on Chr 11, Nnc2 maps to 15 cM
on Chr 14, Nnc3 maps to 82 cM on Chr 1, and Nnc4 maps to 41.5
cM on Chr 16.
Candidates
The significantly reduced ganglion cell number in mice carrying a null
Thra transgene compared to the wildtype supports the candidate gene
Thra for Nnc1. Thra maps within 1–2 cM of Nnc1 on
chromosome 11 (Montgomery et al., 1997), and is expressed within the
developing chick retina (Sjoberg et al., 1992). The ligand of Thra,
triiodothyronine, is known to influence retinal ganglion cell fate
determination (Hoskins, 1985), retinal maturation rate (Macaione et al.,
1984). Hypothyroidism during retinal development results in decreased cell
density in the ganglion cell layer (Hoskins, 1985; Navagantes et al., 1996).
Interestingly, the addition of the Thra ligand, triiodothyronine, to
cultured fetal rat hypothalamus cells stimulates the release IGF-1, a known
mitogen in retinal cultures (Binoux et al., 1985). A candidate gene for
Nnc2 would map on Chr 14 between 11 cM and 17 cM, preferably near the
peak linkage statistic at 15 cM. Two fantastic candidates for Nnc2
are bone morphogenic protein receptor–2/4 (BMPR) and bone
morphogenic protein (BMP). BMPR and BMP map to 13
cM and 14 cM (Beppu et al., 1997). BMPR–2 is a serine–threonine kinase
receptor that is strongly expressed in the developing optic vesicle of the
rat (Obata et al., 1999), and in differentiating ganglion cells in the chick
(Carri et al., 1998). The function of BMPR in the neural retina is
not known, however, in the brain BMPRs are involved in proliferation and
differentiation (Zhang et al., 1998).
The candidate genes for Nnc3 would map on Chr 1 between 77 cM and
88 cM, preferably close to the peak linkage statistic at 82 cM. The best
Nnc3 candidate is retinoid X receptor gamma (Rxrg).
Rxrg maps just within the QTL interval at 88 cM and is expressed in the
developing mouse retina (Dolle et al., 1994). Rxrg null mice appear
normal with respect to growth, behavior, fertility and viability (Krezel et
al., 1996). Interestingly, Rxrg forms a heterodimer with Thra,
the Nnc1 candidate, and together the pair binds to thyroid hormone
response elements to activate gene transcription (Force et al., 1994).
The candidate genes for Nnc4 would map on Chr 16 between 22 cM and
50 cM with the peak association at 41.5 cM. The 2 LOD confidence interval
for this QTL interval is very broad and searching for a candidate gene
within this broad an interval is essentially an exercise in optimism.
However, an attractive candidate does map within the interval at 27 cM, and
this gene is the enhancer of split homolog–1 (HES1). HES1
is basic helix-loop-helix (bHLH) transcription factor expressed in
progenitor cells of the developing neural retina (Kageyama et al., 1997).
Interestingly, HES1 is involved in the Notch pathway, where it acts
downstream of Notch to inhibit neural differentiation. In mice carrying a
null HES1 transgene the retina differentiates prematurely and forms
abnormal rosette structures (Tomita et al., 1996). Genetic variants in
HES1 could alter the expression level or timing of HES1 and this
would affect the number of retinal progenitors produced.
Return to Table of Contents
|
|
|